Distinguished Professor Hua Guo solves 30-year ozone mystery
A group of researchers from the University of New Mexico Department of Chemistry and Chemical Biology, led by Distinguished Professor Hua Guo, have teamed up with colleagues from Texas A&M University to understand more about the photochemistry of Earth’s ozone. A paper authored by the group and recently published in the Proceedings of the National Academy of Sciences of the United States of America (PNAS) offers a long-awaited explanation for the “odd” behavior of ozone molecules in our atmosphere. The discovery was made using quantum mechanical calculations performed with Center for Advanced Research Computing resources.
Ozone (O3) is a gas known for its ability to absorb the sun’s potentially harmful ultraviolet rays in the stratosphere. It performs this function both by splitting up (into a single oxygen atom and an O2 oxygen molecule) and by subsequent recombining. This process also affects air pollution in the lower atmosphere. As Guo explains, “The O atom is largely formed in its excited electronic state and it can have a large impact on the formation of the hydroxyl radical (OH), which serves as the detergent in cleaning various pollutions in Earth’s atmosphere.”
Guo’s paper addresses an intriguing observation about ozone behavior that has puzzled chemists for the past 30 years. Guo writes, “About 30 years ago, an experimental study revealed a strange behavior of the O2 molecule as a result of the breaking-up of ozone. The O2 photofragment was shown to have a strong preference to its rotational states with even quantum numbers, while the rotational states with odd quantum numbers are much less populated. Here, the rotational quantum number dictates how fast the O2 molecule rotates and it contains important information about how the O3 molecules break up in an excited electronic state.”
To study this phenomenon, Guo’s team performed extensive calculations using quantum mechanical theory. Their work solves the Schrödinger equation, a differential equation of central importance to quantum mechanics. Because of the immense complexity of these calculations, Guo’s team used the computing resources at CARC to complete the project. “We solved the Schrödinger equation to understand the quantum mechanics of the ozone dissociation. The Schrödinger equation is a differential equation and its solution can only be obtained with the aid of a supercomputer,” Guo comments.
The results of this research definitively explain why the O2 photofragment disproportionately favors rotational states with even quantum numbers. According to Guo, “the even-odd propensity in the O2 rotational states stems from preference of O3 to break up within the molecular plane.” Coauthor Shanyu Han, who is a postdoc in Guo’s group, adds, “When one chemical bond of the ozone is broken and oxygen is formed, one ‘half’ of oxygen is filtered, and the other ‘half’ is enhanced. And our finding is that the ‘filter’ turns out to be the symmetry of the oxygen molecule and the bosonic nature of oxygen atom, which is exactly the consequence of quantum mechanics.”
While plans for follow-up research have not been made at this time, Guo and Han continue to expand chemistry literature with their computational work. A paper coauthored by Guo, titled “Following the microscopic pathway to adsorption through chemisorption and physisorption wells,” was published by Science in September 2020, while a paper coauthored by Han, “Impact of Diabolical Singular Points on Nonadiabatic Dynamics and A Remedy: Photodissociation of Ammonia in the First Band,” was published by the Journal of Chemical Theory and Computation in October 2020. Both papers were based on computation performed using CARC systems.
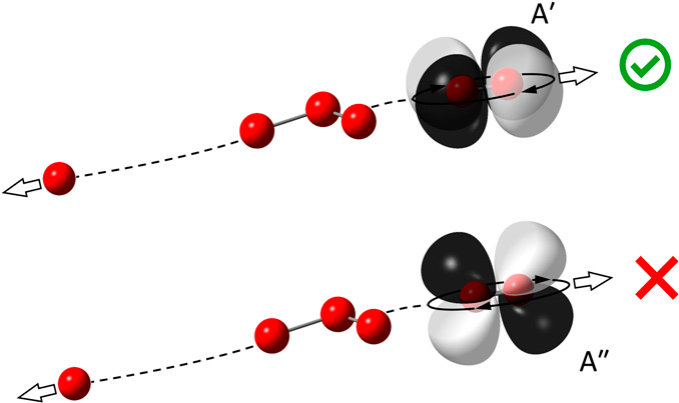
An illustration of quantum states of an ozone molecule, courtesy of Dr. Hua Guo